Take control of your heart health with our intuitive heart rate monitoring solution
A
A
Hardware Overview
How does it work?
Heart Rate 2 Click is based on MAXM86161, a complete, integrated optical data acquisition system from Analog Devices ideal for optical pulse oximetry and heart-rate detection applications. It comprises a single optical readout channel and current LED driver DACs that modulate LED pulses for various optical measurements. The LED current DACs have 8 bits of dynamic range with four programmable full-scale ranges of 31mA, 62mA, 94mA, and 124mA. The LED drivers are low dropout current sources allowing for low-noise, power-supply independent LED currents to be sourced at the lowest supply voltage possible, minimizing LED power consumption. The LED pulse width can be programmed from 14.8μs to 117.3μs to allow the algorithms to optimize SpO2 and HR accuracy at the lowest dynamic power consumption dictated by the application. The optical subsystem Heart Rate 2 Click based on MAXM86161 comprises ambient light cancellation (ALC), a continuous-time
sigma-delta ADC, and a proprietary discrete time filter. ALC incorporates a proprietary scheme to cancel ambient-light-generated photodiode currents, allowing the sensor to work in high ambient light conditions. The optical ADC has programmable full-scale ranges of 4μA to 32μA. The internal ADC is a continuous time, oversampling sigma-delta converter with 19-bit resolution. The ADC output data rate can be programmed from 8sps (samples per second) to 4096sps. The MAXM86161 includes a proprietary discrete time filter to reject 50Hz/60Hz interference and change residual ambient light from the sensor measurements. The sensor also includes an optical proximity function which could significantly reduce energy consumption and extend battery life when the sensor is not in contact with the skin. MAXM86161 power to a minimum during situations with no reflective returned signal. It is also intended to reduce the emitted light to a minimum or even below
what the human eye perceives. The native delta-sigma ADC linearity is exceptional. However, the sub-ranging DAC uses a unary architecture with some mismatch between the DAC's unit current sources and the ADC reference current. This mismatch results in some transfer function nonlinearity (XNL) errors when the sub-DAC code transitions. For this reason, the sub-ranging DAC algorithm is designed to minimize DAC transitions by introducing large hysteresis through the overlapping sub-DAC ranges. Consequently, under normal PPG operation, the sub-DAC does not transition, and the linearity of the converter signal is driven entirely by the linear native delta-sigma ADC. In addition to algorithmically reducing the sub-DAC transitions, the MAXM86161 incorporates a self-calibration scheme that can be used to reduce the sub-DAC XNL errors further.
Features overview
Development board
Nucleo-64 with STM32F091RC MCU offers a cost-effective and adaptable platform for developers to explore new ideas and prototype their designs. This board harnesses the versatility of the STM32 microcontroller, enabling users to select the optimal balance of performance and power consumption for their projects. It accommodates the STM32 microcontroller in the LQFP64 package and includes essential components such as a user LED, which doubles as an ARDUINO® signal, alongside user and reset push-buttons, and a 32.768kHz crystal oscillator for precise timing operations. Designed with expansion and flexibility in mind, the Nucleo-64 board features an ARDUINO® Uno V3 expansion connector and ST morpho extension pin
headers, granting complete access to the STM32's I/Os for comprehensive project integration. Power supply options are adaptable, supporting ST-LINK USB VBUS or external power sources, ensuring adaptability in various development environments. The board also has an on-board ST-LINK debugger/programmer with USB re-enumeration capability, simplifying the programming and debugging process. Moreover, the board is designed to simplify advanced development with its external SMPS for efficient Vcore logic supply, support for USB Device full speed or USB SNK/UFP full speed, and built-in cryptographic features, enhancing both the power efficiency and security of projects. Additional connectivity is
provided through dedicated connectors for external SMPS experimentation, a USB connector for the ST-LINK, and a MIPI® debug connector, expanding the possibilities for hardware interfacing and experimentation. Developers will find extensive support through comprehensive free software libraries and examples, courtesy of the STM32Cube MCU Package. This, combined with compatibility with a wide array of Integrated Development Environments (IDEs), including IAR Embedded Workbench®, MDK-ARM, and STM32CubeIDE, ensures a smooth and efficient development experience, allowing users to fully leverage the capabilities of the Nucleo-64 board in their projects.
Microcontroller Overview
MCU Card / MCU
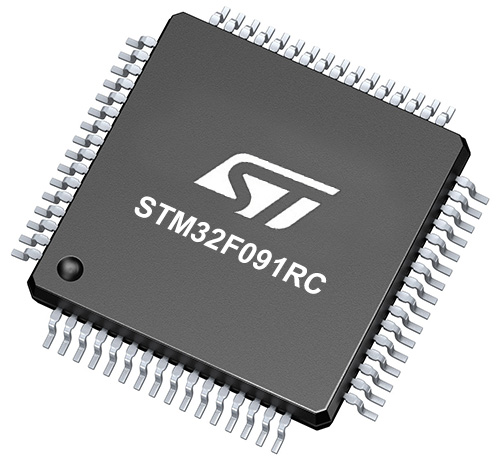
Architecture
ARM Cortex-M0
MCU Memory (KB)
256
Silicon Vendor
STMicroelectronics
Pin count
64
RAM (Bytes)
32768
You complete me!
Accessories
Click Shield for Nucleo-64 comes equipped with two proprietary mikroBUS™ sockets, allowing all the Click board™ devices to be interfaced with the STM32 Nucleo-64 board with no effort. This way, Mikroe allows its users to add any functionality from our ever-growing range of Click boards™, such as WiFi, GSM, GPS, Bluetooth, ZigBee, environmental sensors, LEDs, speech recognition, motor control, movement sensors, and many more. More than 1537 Click boards™, which can be stacked and integrated, are at your disposal. The STM32 Nucleo-64 boards are based on the microcontrollers in 64-pin packages, a 32-bit MCU with an ARM Cortex M4 processor operating at 84MHz, 512Kb Flash, and 96KB SRAM, divided into two regions where the top section represents the ST-Link/V2 debugger and programmer while the bottom section of the board is an actual development board. These boards are controlled and powered conveniently through a USB connection to program and efficiently debug the Nucleo-64 board out of the box, with an additional USB cable connected to the USB mini port on the board. Most of the STM32 microcontroller pins are brought to the IO pins on the left and right edge of the board, which are then connected to two existing mikroBUS™ sockets. This Click Shield also has several switches that perform functions such as selecting the logic levels of analog signals on mikroBUS™ sockets and selecting logic voltage levels of the mikroBUS™ sockets themselves. Besides, the user is offered the possibility of using any Click board™ with the help of existing bidirectional level-shifting voltage translators, regardless of whether the Click board™ operates at a 3.3V or 5V logic voltage level. Once you connect the STM32 Nucleo-64 board with our Click Shield for Nucleo-64, you can access hundreds of Click boards™, working with 3.3V or 5V logic voltage levels.
Used MCU Pins
mikroBUS™ mapper
Take a closer look
Click board™ Schematic
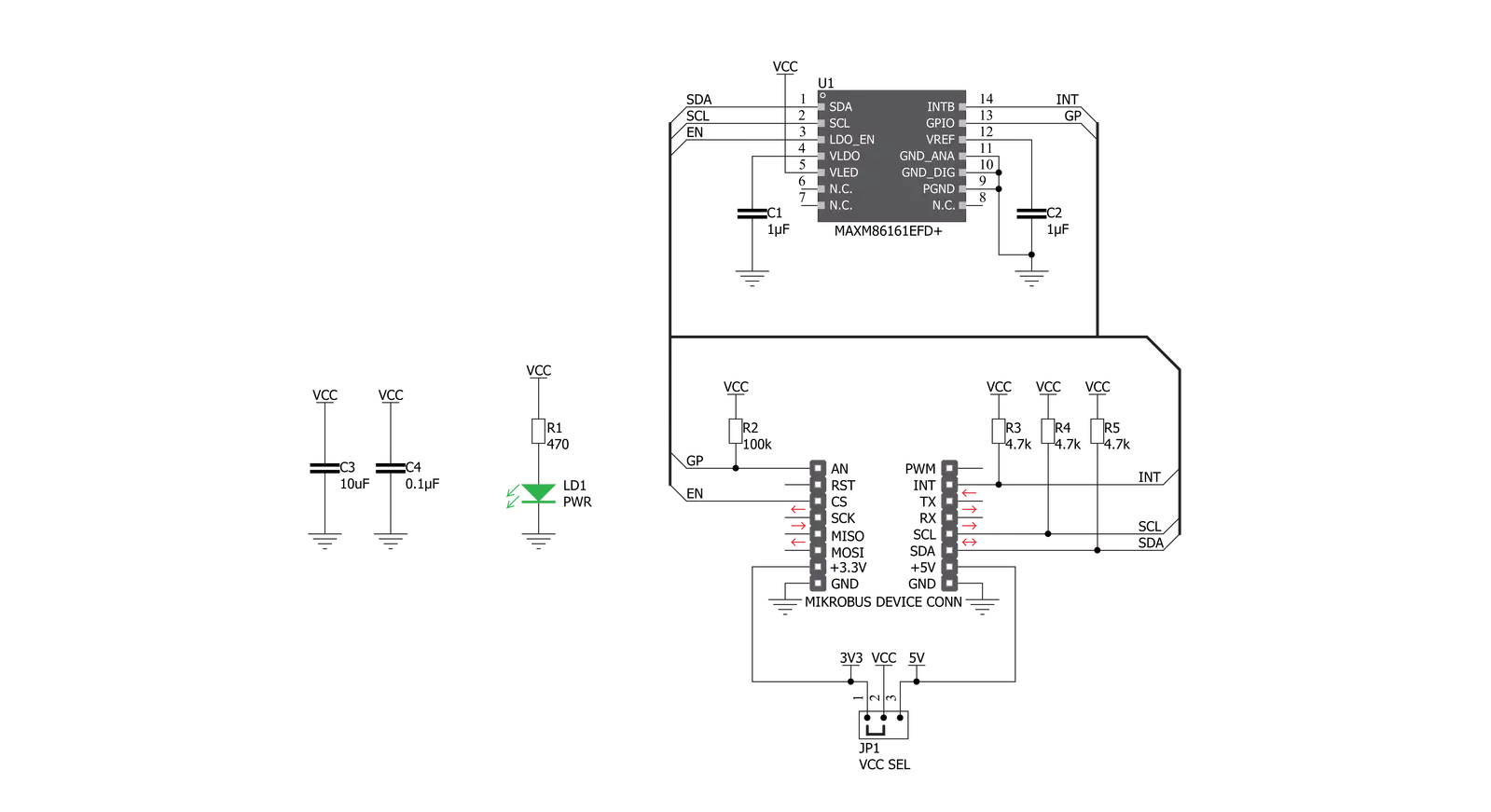
Step by step
Project assembly
Track your results in real time
Application Output
1. Application Output - In Debug mode, the 'Application Output' window enables real-time data monitoring, offering direct insight into execution results. Ensure proper data display by configuring the environment correctly using the provided tutorial.
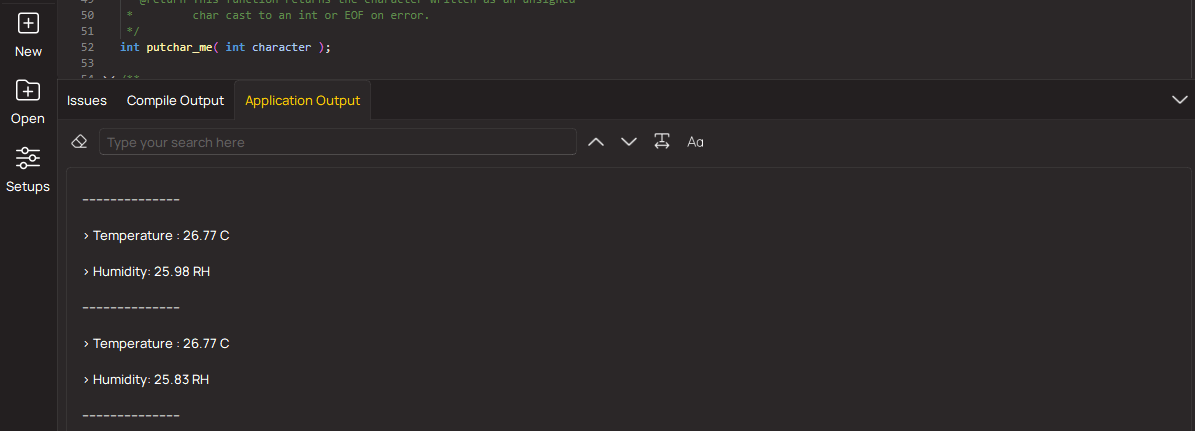
2. UART Terminal - Use the UART Terminal to monitor data transmission via a USB to UART converter, allowing direct communication between the Click board™ and your development system. Configure the baud rate and other serial settings according to your project's requirements to ensure proper functionality. For step-by-step setup instructions, refer to the provided tutorial.
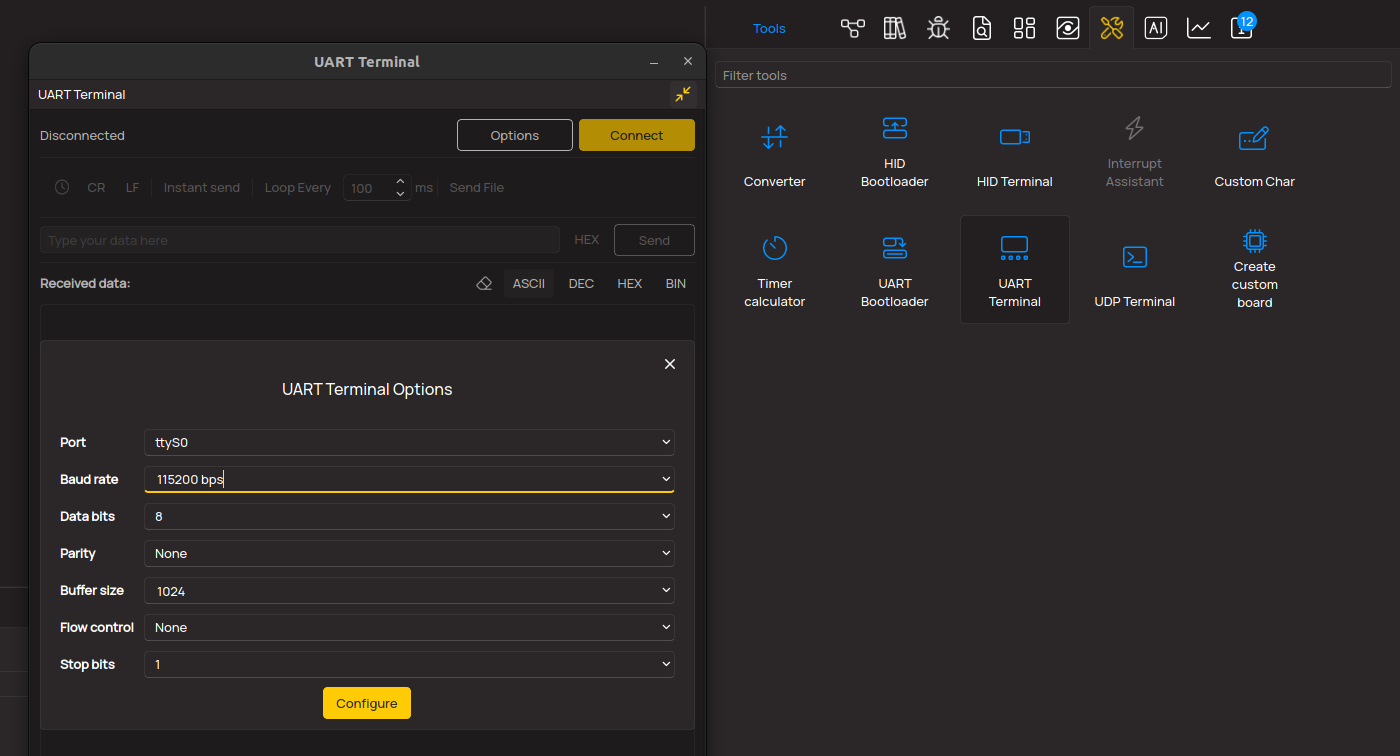
3. Plot Output - The Plot feature offers a powerful way to visualize real-time sensor data, enabling trend analysis, debugging, and comparison of multiple data points. To set it up correctly, follow the provided tutorial, which includes a step-by-step example of using the Plot feature to display Click board™ readings. To use the Plot feature in your code, use the function: plot(*insert_graph_name*, variable_name);. This is a general format, and it is up to the user to replace 'insert_graph_name' with the actual graph name and 'variable_name' with the parameter to be displayed.
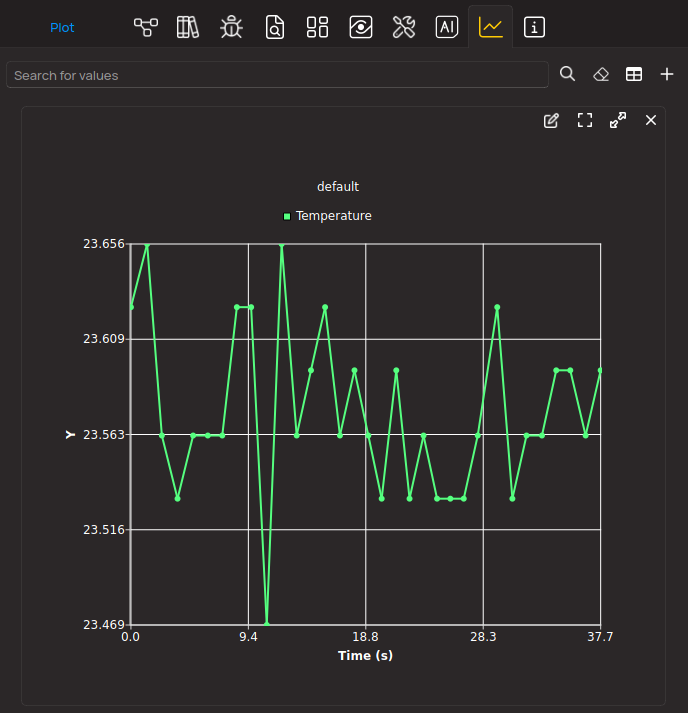
Software Support
Library Description
This library contains API for Heart Rate 2 Click driver.
Key functions:
uint8_t heartrate2_generic_read ( uint8_t reg_adr )
- Generic function for reading data.void heartrate2_generic_write ( uint8_t reg_adr, uint8_t write_data )
- Generic function for writing data.void heartrate2_default_configuration ( uint8_t cfg_led )
- Function for setting default config and turning on one led with cfg_led parameter.void heartrate2_read_fifo ( heartrate2_fifo_data_t *fifo )
- Function for reading fifo data and tag values from device.
Open Source
Code example
The complete application code and a ready-to-use project are available through the NECTO Studio Package Manager for direct installation in the NECTO Studio. The application code can also be found on the MIKROE GitHub account.
/*!
* \file
* \brief HeartRate2 Click example
*
* # Description
* This example demonstrates the use of Heart rate 2 click board.
*
* The demo application is composed of two sections :
*
* ## Application Init
* Initilizes the driver, resets the device, checks the device ID and applies default settings.
*
* ## Application Task
* Reads the data from Green diode and displays the results on USB UART if the measured data
* is above defined threshold, otherwise, it displays a desired message on the terminal.
*
* \author MikroE Team
*
*/
// ------------------------------------------------------------------- INCLUDES
#include "board.h"
#include "log.h"
#include "heartrate2.h"
// ------------------------------------------------------------------ VARIABLES
static heartrate2_t heartrate2;
static log_t logger;
static uint16_t counter = 1000;
// ------------------------------------------------------ APPLICATION FUNCTIONS
void application_init ( void )
{
log_cfg_t log_cfg;
heartrate2_cfg_t cfg;
uint8_t rd_stat;
/**
* Logger initialization.
* Default baud rate: 115200
* Default log level: LOG_LEVEL_DEBUG
* @note If USB_UART_RX and USB_UART_TX
* are defined as HAL_PIN_NC, you will
* need to define them manually for log to work.
* See @b LOG_MAP_USB_UART macro definition for detailed explanation.
*/
LOG_MAP_USB_UART( log_cfg );
log_init( &logger, &log_cfg );
log_info( &logger, "---- Application Init ----" );
// Click initialization.
heartrate2_cfg_setup( &cfg );
HEARTRATE2_MAP_MIKROBUS( cfg, MIKROBUS_1 );
heartrate2_init( &heartrate2, &cfg );
log_printf( &logger, "Configuring the module...\r\n" );
Delay_ms ( 1000 );
heartrate2_set_en( &heartrate2, HEARTRATE2_PIN_HIGH );
Delay_ms ( 100 );
heartrate2_soft_reset ( &heartrate2 );
rd_stat = heartrate2_generic_read( &heartrate2, HEARTRATE2_REG_PART_ID );
if ( rd_stat != HEARTRATE2_DEV_ID )
{
log_error( &logger, "---- WRONG ID ----" );
log_printf( &logger, "Please restart your system.\r\n" );
for ( ; ; );
}
heartrate2_default_cfg( &heartrate2, HEARTRATE2_CONFIG_GREEN );
log_printf( &logger, "The module has been configured!\r\n" );
Delay_ms ( 1000 );
}
void application_task ( void )
{
heartrate2_fifo_data_t fifo_object;
heartrate2_read_fifo( &heartrate2, &fifo_object );
if ( fifo_object.tag == HEARTRATE2_FIFO_TAG_PPG1_LEDC1 )
{
counter++;
if ( fifo_object.data_val > 1000 )
{
log_printf( &logger, "%lu;\r\n", fifo_object.data_val );
counter = 1000;
}
else if ( counter > 1000 )
{
log_printf( &logger, "Please place your index finger on the sensor.\r\n" );
counter = 0;
}
}
}
int main ( void )
{
/* Do not remove this line or clock might not be set correctly. */
#ifdef PREINIT_SUPPORTED
preinit();
#endif
application_init( );
for ( ; ; )
{
application_task( );
}
return 0;
}
// ------------------------------------------------------------------------ END